Home » Student Resources » Online Chemistry Textbooks » CH105: Consumer Chemistry » CH105: Chapter 9 – Organic Compounds of Oxygen
MenuCH105: Consumer Chemistry
Chapter 9 – Organic Compounds of Oxygen
Opening Essay
9.1 Introduction to Compounds that Contain Oxygen
9.2 Alcohols and Phenols
Classification of Alcohols
Properties of Alcohols
Glycols
Phenols
9.3 Ethers
Properties of Ethers
9.4 Aldehydes and Ketones
Properties of Aldehydes and Ketones
Aldehydes
Ketones
Boiling Points and Solubility
Aldehydes and Ketones in Nature
9.5 Carboxylic Acids and Esters
Properties of Carboxylic Acids and Esters
Carboxylic Acids
Esters
Boiling Points, Melting Points and Solubility
Commonly Used Carboxylic Acids and Esters
9.6 Reactions of Oxygen-Containing Compounds
Alcohols
Dehydration (Elimination) Reactions
Oxidation Reactions
Aldehydes and Ketones
Oxidation Reactions
Reduction Reactions
Addition Reactions with Alcohols (Hemiacetals and Hemiketals)
Reactions that form Acetals or Ketals
Carboxylic Acids
The Acidity of Carboxylic Acids
Carboxylic Acids and the Formation of Salts
Formation of Esters from Carboxylic Acids and Alcohols
Hydrolysis of Esters
9.7 Chapter Summary
9.8 References
Opening Essay
Photo By: A. Savin
9.1 Introduction to Compounds that Contain Oxygen
In this chapter you will be introduced to the major organic functional groups that contain oxygen. This includes alcohols, phenols, ethers, aldehydes, ketones, carboxylic acids, and esters. Figure 9.1 provides the basic organic functional groups for these compounds and the IUPAC suffix that is used to name these compounds. While you will not have to formally name complete structures, you should be able to identify functional groups contained within compounds based on their IUPAC names. For example, an alcohol is an organic compound with a hydroxyl (-OH) functional group on an aliphatic carbon atom. Because -OH is the functional group of all alcohols, we often represent alcohols by the general formula ROH, where R is an alkyl group. The IUPAC nomenclature guidelines use the suffix ‘-ol’ to denote simple compounds that contain alcohols. An example is ethanol (CH3CH2OH).
Figure 9.1 Common Organic Functional Groups that Contain Oxygen. The IUPAC suffixes used in naming simple organic molecules are noted in the chart
9.2 Alcohols and Phenols
Classification of Alcohols
Some of the properties and reactivity of alcohols depend on the number of carbon atoms attached to the specific carbon atom that is attached to the -OH group. Alcohols can be grouped into three classes on this basis.
- A primary (1°) alcohol is one in which the carbon atom (in red) with the OH group is attached to one other carbon atom (in blue). Its general formula is RCH2OH.

- A secondary (2°) alcohol is one in which the carbon atom (in red) with the OH group is attached to two other carbon atoms (in blue). Its general formula is R2CHOH.

- A tertiary (3°) alcohol is one in which the carbon atom (in red) with the OH group is attached to three other carbon atoms (in blue). Its general formula is R3COH.

Properties of Alcohols
Alcohols can be considered derivatives of water (H2O; also written as HOH).

Like the H–O–H bond in water, the R–O–H bond is bent, and the -OH portion of alcohol molecules are polar. This relationship is particularly apparent in small molecules and reflected in the physical and chemical properties of alcohols with low molar mass. Replacing a hydrogen atom from an alkane with an OH group allows the molecules to associate through hydrogen bonding (Figure 9.2).
Figure 9.2 Intermolecular Hydrogen Bonding in Methanol. The OH groups of alcohol molecules make hydrogen bonding possible.
Recall that physical properties are determined to a large extent by the type of intermolecular forces. Table 9.1 lists the molar masses and the boiling points of some common compounds. The table shows that substances with similar molar masses can have quite different boiling points.
Table 9.1 Comparison of Molar Mass and Boiling Points
Alkanes are nonpolar and are thus associated only through relatively weak London Dispersion Forces (LDFs). The boiling points of alkanes with one to four carbon atoms are so low that all of these molecules are gases at room temperature. In contrast, if we analyze the compounds that contain an alcohol functional group, even methanol (with only one carbon atom) is a liquid at room temperature. Since alcohols have the capacity to form hydrogen bonds, their boiling points are significantly higher when compared to hydrocarbons of comparable molar mass. The boiling point is a rough measure of the amount of energy necessary to separate a liquid molecule from its nearest neighbors. If the molecules interact through hydrogen bonding, a relatively large quantity of energy must be supplied to break those intermolecular attractions. Only then can the molecule escape from the liquid into the gaseous state.
Another interesting trend is apparent in table 9.1, is that as the alcohol molecules have more carbons, they also have higher boiling points. This is because molecules may have more than one type of intermolecular interactions. In addition to hydrogen bonding, alcohol molecules also have LDFs that occur between the nonpolar portions of the molecules. As we saw with the alkanes, the larger the carbon chain, the more LDFs that are present within the molecule. As with the alkanes, an increased amount of LDFs in alcohol containing molecules also causes in increase in boiling point.
In addition to forming hydrogen bonds with themselves, alcohols can also engage in hydrogen bonding with water molecules (Figure 9.3). Thus, whereas the hydrocarbons are insoluble in water, small alcohols with one to three carbon atoms are completely soluble. As the length of the chain increases, however, the solubility of alcohols in water decreases; the molecules become more like hydrocarbons and less like water. The alcohol 1-decanol (CH3CH2CH2CH2CH2CH2CH2CH2CH2CH2OH) that contains 10 carbon atoms is essentially insoluble in water. We frequently find that the borderline of solubility in a family of organic compounds occurs at four or five carbon atoms.
Figure 9.3 Hydrogen Bonding between Methanol Molecules and Water Molecules. Hydrogen bonding between the OH of methanol and water molecules accounts for the solubility of methanol in water.
Glycols
Molecules that contain two alcohol functional groups are often called glycols. Ethylene glycol, one of the simplest glycols, has two major commercial uses. It is used as a raw material in the manufacture of polyester fibers and for antifreeze formulations. The addition of two or more -OH groups to a hydrocarbon substantially increases the boiling point and solubility of the alcohol. For example, for ethylene glycol, the boiling point is 197.3oC, compared to ethanol which has a boiling point of 78oC. Thus, ethylene glycol is a useful cooling substance for automobile engines.
Figure 9.4 Properties of Ethylene Glycol. Ethylene glycol is often used as a cooling agent in antifreeze mixtures due to its low freezing point and high boiling point.
Ethylene glycol is poisonous to humans and other animals, and should be handled carefully and disposed of properly. As a clear liquid with a sweet taste, it can lead to accidental ingestion, especially by pets, or it can be used deliberately as a murder weapon. Ethylene glycol is difficult to detect in the body, and causes symptoms—including intoxication, severe diarrhea, and vomiting—that can be confused with other illnesses or diseases. Its metabolism produces calcium oxalate, which crystallizes in the brain, heart, lungs, and kidneys, damaging them; depending on the level of exposure, accumulation of the poison in the body can last weeks or months before causing death, but death by acute kidney failure can result within 72 hours if the individual does not receive appropriate medical treatment for the poisoning. Some ethylene glycol antifreeze mixtures contain an embittering agent, such as denatonium, to discourage accidental or deliberate consumption. Typical antifreeze mixtures also contain a fluorescent green dye which make it easier to find and clean up antifreeze spills.
Phenols
Compounds in which an -OH group is attached directly to an aromatic ring are called phenols and can be abbreviated ArOH in chemical equations. Phenols differ from alcohols in that they are slightly acidic in water. Similar to double displacement acid-base neutralization reactions, they react with aqueous sodium hydroxide (NaOH) to form a salt and water.
The simplest phenol containing compound, C6H5OH, is itself called phenol. (An older name, emphasizing its slight acidity, was carbolic acid.) Phenol is a white crystalline compound that has a distinctive (“hospital smell”) odor.
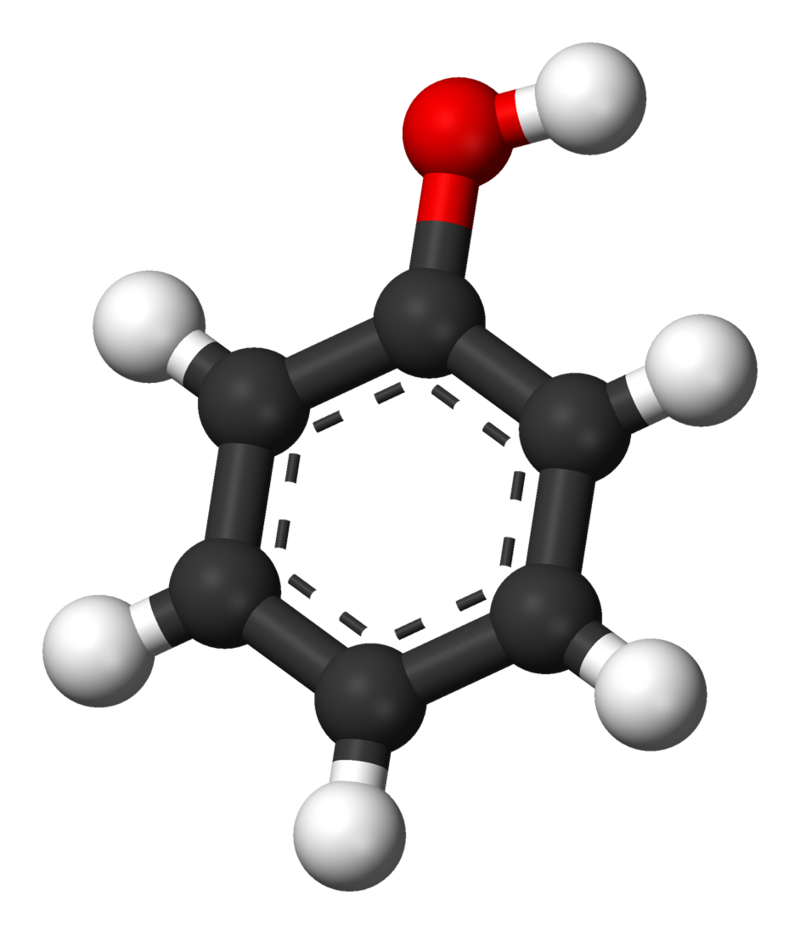
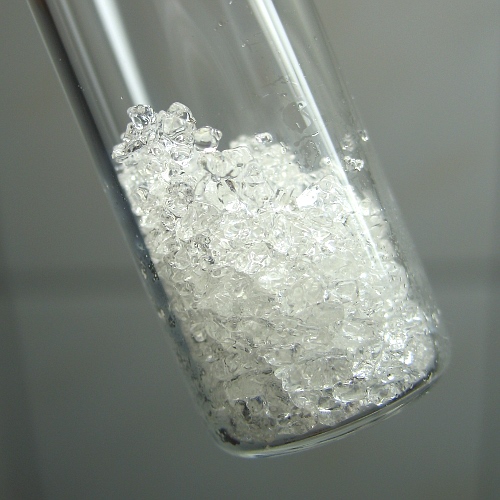
To Your Health: Phenols and Us
Phenols are widely used as antiseptics (substances that kill microorganisms on living tissue) and as disinfectants (substances intended to kill microorganisms on inanimate objects such as furniture or floors). The first widely used antiseptic was phenol. Joseph Lister used it for antiseptic surgery in 1867. Phenol is toxic to humans, however, and can cause severe burns when applied to the skin. In the bloodstream, it is a systemic poison, meaning that it is carried to and affects all parts of the body. Its severe side effects led to searches for safer antiseptics, a number of which have been found.
Figure 9.6 An operation in 1753 of a surgery before antiseptics were used. Picture is painted by Gaspare Traversi.
Currently, phenol is only used in very small concentrations in some over-the-counter medical products like chloraseptic throat spray.
Figure 9.7 Phenol is still used in low concentrations in some medical formulations such as chloraseptic.
More complex compounds that contain phenolic functional groups are commonly found in nature, especially as plant natural products. For example, some of the major metabolites found in green tea are the polyphenolic catechin compounds, represented in figure 9.8A by epigallocatechin gallate (ECGC) and epicatechin. Drinking green tea has been shown to have chemopreventative properties in laboratory animals. The biological activity of the catechins as antioxidant agents is thought to contribute to this activity and other health benefits attributed to tea consumption. Some of the biologically active constituents of marijuana, such as tetrahydrocannabinol (THC) and cannabidiol (CBD) are also phenolic compounds (Fig 9B).
Figure 9.8 Plant-derived natural products that contain phenolic functional groups. (A) Green tea contains catechin compounds like epigallocatechin gallate (ECGC) and the epicatechins that are thought to provide some of the anticancer health benefits attributed to green tea. (B) Marijuana contains many biologically active phenolic compounds, including the hallucinogenic component of marijuana, tetrahydrocannabinol (THC) and the metabolite cannabidiol (CBD). Cannabidiol does not have psychoactive properties and is currently being studied as a potential medical treatment for refractive epilepsy syndromes.
Concept Review Exercises
-
Why is ethanol (CH3CH2OH) more soluble in water than 1-hexanol (CH3CH2CH2CH2CH2CH2OH)?
-
Why does 1-butanol (CH3CH2CH2CH2OH) have a lower boiling point than 1-hexanol (CH3CH2CH2CH2CH2CH2OH)?
Answers
-
Ethanol has an -OH group and only 2 carbon atoms; 1-hexanol has one -OH group for 6 carbon atoms and is thus more like a (nonpolar) hydrocarbon than ethanol.
-
1-hexanol has a longer carbon chain than that of 1-butanol and therefore more LDFs that contribute to a higher boiling point.
Exercises
Answer the following exercises without consulting tables in the text.
-
Arrange these alcohols in order of increasing boiling point: 1-butanol (CH3CH2CH2CH2OH), 1-heptanol (CH3CH2CH2CH2CH2CH2CH2OH), and 1-propanol (CH3CH2CH2OH).
-
Which has the higher boiling point—butane (CH3CH2CH2CH3) or 1-propanol (CH3CH2CH2OH)?
-
Arrange these alcohols in order of increasing solubility in water: 1-butanol (CH3CH2CH2CH2OH), methanol (CH3OH), and 1-octanol (CH3CH2CH2CH2CH2CH2CH2CH2OH).
-
Arrange these compounds in order of increasing solubility in water: 1-butanol (CH3CH2CH2CH2OH), ethanol (CH3CH2OH), and pentane (CH3CH2CH2CH2CH3).
Answers to Odd Questions
-
1-propanol < 1-butanol < 1-heptanol
-
1-octanol < 1-butanol < methanol
9.3 Ethers
Ethers are a class of organic compounds that contain an oxygen between two alkyl groups. They have the formula R-O-R’, with R’s being the alkyl groups. these compounds are used in dye, perfumes, oils, waxes and industrial use.
Properties of Ethers
The C – O bonds in ethers are polar and thus ethers have a net dipole moment. The weak polarity of ethers do not appreciably affect their boiling points which are comparable to those of the alkenes of comparable molecular mass. Ethers have much lower boiling points as compared to isomeric alcohols. This is because alcohols molecules are associated by hydrogen bonds while ether molecules cannot form hydrogen bonds with other ether molecules. For example, diethyl ether (CH3CH2OCH2CH3) has a boiling point of 34.6oC whereas n-butanol, (CH3CH2CH2CH2OH), a four carbon alcohol, has a boiling piont of 117.7oC.
Ethers can form hydrogen bonds with water, however, as water contains the partially positive hydrogen atoms required for H-bonding. Thus, ethers containing up to 3 carbon atoms are soluble in water, due to the formation of H-bonds with water molecules.
The solubility of ethers decreases with an increase in the number of carbon atoms. The relative increase in the hydrocarbon portion of the molecule decreases the tendency of H-bond formation with water. Ethers are appreciably soluble in more nonpolar organic solvents and in fact, can be used as a solvent to dissolve nonpolar to mildly polar molecules. In addition, ethers are very non-reactive. In fact, with the exception of the alkanes, cycloalkanes and fluorocarbons, ethers are probably the least reactive common class of organic compounds. Thus, smaller ethers, like diethyl ether (CH3CH2OCH2CH3) are widely used by organic chemists as solvents for a variety of organic reactions. The inert nature of the ethers relative to the alcohols is undoubtedly due to the absence of the reactive O–H bond.
To Your Health – Ethers and Us
In the mid 1800’s and early 1900’s diethyl ether was used as an anesthetic during surgery, largely replacing chloroform due to reduced toxicity. A general anesthetic acts on the brain to produce unconsciousness and a general insensitivity to feeling or pain. Diethyl ether (CH3CH2OCH2CH3) was the first general anesthetic to be commonly used.
Figure 9.9 William Morton, a Boston dentist, introduced diethyl ether into surgical practice in 1846. This painting shows an operation in Boston in 1846 in which diethyl ether was used as an anesthetic. Inhalation of ether vapor produces unconsciousness by depressing the activity of the central nervous system. Source: Painting of William Morton by Ernest Board.
Diethyl ether is relatively safe because there is a fairly wide gap between the dose that produces an effective level of anesthesia and the lethal dose. However, because it is highly flammable and has the added disadvantage of causing nausea, it has been replaced by newer inhalant anesthetics, including the fluorine-containing compounds halothane, and the halogenated ethers, desflurane, isoflurane, and sevoflurane. The halogenated ethers, isoflurane, desflurane, and sevoflurane, show reduced side effects when compared with diethyl ether. Unfortunately, the safety of these compounds for operating room personnel has been questioned. For example, female operating room workers exposed to halothane suffer a higher rate of miscarriages than women in the general population.
Ethers are also common functional groups found in natural products and can have unique biological activities. In fact, some very large compounds containing multiple ethers, called polyethers, have been found to cause neurotoxic shellfish poisoning. In this example, the dinoflaggelate, Karina brevis, which is the causative agent of red tide algal blooms, produces a class of highly toxic polyethers called the brevatoxins. Brevatoxin A is depicted in Figure 9.10. Symptoms of this poisoning include vomiting and nausea and a variety of neurological symptoms such as slurred speech.
Figure 9.10 Neurotoxic Shellfish Poisoning. The dinoflaggelate, Karina brevis, shown in the upper left is the causative agent of red tide harmful algal blooms. These marine algal blooms can be quite extensive as shown in the photo of a red tide (upper right) occurring near San Diego, CA. K. brevis produces a class of polyethers called the brevatoxins. Brevatoxin A is depicted as an example. Filter feeding clams and muscles become contaminated with the dinoflaggelate and can cause neurotoxic shellfish poisoning if eaten. Red tides can have severe economic costs as fisheries and shellfish harvesting has to be closed until toxin levels in commercial products return to acceptable levels.
9.4 Aldehydes and Ketones
Aldehydes and ketones are characterized by the presence of a carbonyl group (C=O), and their reactivity can generally be understood by recognizing that the carbonyl carbon contains a partial positive charge (δ+) and the carbonyl oxygen contains a partial negative charge (δ−). Aldehydes are typically more reactive than ketones.
The Carbonyl Group
A carbonyl group is a chemically organic functional group composed of a carbon atom double-bonded to an oxygen atom –> [C=O] The simplest carbonyl groups are aldehydes and ketones usually attached to another carbon compound. These structures can be found in many aromatic compounds contributing to smell and taste.
Before going into anything in depth be sure to understand that the C=O entity itself is known as the “carbonyl group” while the members of this group are called “carbonyl compounds“.
As discussed before, we understand that oxygen has two lone pairs of electrons hanging around. These electrons make the oxygen more electronegative than carbon. The carbon is then partially positive (or electrophillic = ‘electron loving’) and the oxygen partially negative (nucleophillic = ‘nucleus or proton loving’). The polarizability is denoted by a lowercase delta and a positive or negative superscript depending on the atom. For example, carbon would have δ+ and oxygen δ–.
Properties of Aldehydes and Ketones
Aldehydes
In aldehydes, the carbonyl group has a hydrogen atom attached to it together with either
- a second hydrogen atom
- or, more commonly, a hydrocarbon group which might be an alkyl group or one containing a benzene ring.
For the purposes of this section, we shall ignore those containing benzene rings. Below are some examples of aldehydes
Notice that these all have exactly the same end to the molecule. All that differs is the complexity of the other carbon group attached. When you are writing formulae for these, the aldehyde group (the carbonyl group with the hydrogen atom attached) is always written as -CHO – never as COH. That could easily be confused with an alcohol. Ethanal, for example, is written as CH3CHO; methanal as HCHO.
Ketones
In ketones, the carbonyl group has two carbon groups attached. Again, these can be either alkyl groups or ones containing benzene rings. Notice that ketones never have a hydrogen atom attached to the carbonyl group.
Propanone is normally written CH3COCH3.
Boiling Points and Solubility
Methanal, also known as formaldehyde, (HCHO) is a gas at room temperature (boiling point -21°C), and ethanal, also known as acetaldehyde, has a boiling point of +21°C. That means that ethanal boils at close to room temperature. Larger aldehydes and the ketones are liquids, with boiling points rising as the molecules get bigger. The size of the boiling point is governed by the strengths of the intermolecular forces. There are two main intermolecular forces found in these molecules:
- London dispersion forces: These attractions get stronger as the molecules get longer and have more electrons. That increases the sizes of the temporary dipoles that are set up. This is why the boiling points increase as the number of carbon atoms in the chains increases – irrespective of whether you are talking about aldehydes or ketones.
- Dipole-Dipole attractions: Both aldehydes and ketones are polar molecules because of the presence of the carbon-oxygen double bond. As well as the dispersion forces, there will also be attractions between the permanent dipoles on nearby molecules. That means that the boiling points will be higher than those of similarly sized hydrocarbons – which only have dispersion forces. It is interesting to compare three similarly sized molecules. They have similar lengths, and similar (although not identical) numbers of electrons.
The polarization of carbonyl groups also effects the boiling point of aldehydes and ketones which is higher than those of hydrocarbons of similar size. However, since they cannot form hydrogen bonds, their boiling points tend to be lower than alcohols of similar size. Table 9.2 gives some examples of compounds of similar mass, but that contain different types of functional groups. Note that compounds that have stronger intermolecular forces have higher boiling points.
Alkanes < Aldehydes < Ketones < Alcohols
Table 9.2 Comparison of Boiling Points and Intermolecular Forces
Due to the polarity of the carbonyl group, the oxygen atom of the aldehyde or ketone engages in hydrogen bonding with a water molecule.

The solubility of aldehydes and ketones are therefore about the same as that of alcohols and ethers. Formaldehyde (HCHO), acetaldehyde (CH3CHO), and acetone ((CH3)2CO) are soluble in water. As the carbon chain increases in length, solubility in water decreases. The borderline of solubility occurs at about four carbon atoms per oxygen atom. All aldehydes and ketones are soluble in organic solvents and, in general, are less dense than water.
Aldehydes and Ketones in Nature
Similar to the other oxygen-containing functional groups discussed thus far, aldehydes and ketones are also widespread in nature and are often combined with other functional groups. Examples of naturally occurring molecules which contain a aldehyde or ketone functional group are shown in the following two figures. The compounds in the figure 9.11 are found chiefly in plants or microorganisms and those in the figure 9.12 have animal origins. Many of these molecular structures are chiral and have distinct stereochemistry.
When chiral compounds are found in nature they are usually enantiomerically pure, although different sources may yield different enantiomers. For example, carvone is found as its levorotatory (R)-enantiomer in spearmint oil, whereas, caraway seeds contain the dextrorotatory (S)-enantiomer. In this case the change of the stereochemistry causes a drastic change in the perceived scent. Aldehydes and ketones are known for their sweet and sometimes pungent odors. The odor from vanilla extract comes from the molecule vanillin. Likewise, benzaldehyde provides a strong scent of almonds. Because of their pleasant fragrances aldehyde and ketone containing molecules are often found in perfumes. However, not all of the fragrances are pleasing. In particular, 2-Heptanone provides part of the sharp scent from blue cheese and (R)-Muscone is part of the musky smell from the Himalayan musk deer. Lastly, ketones show up in many important hormones such as progesterone (a female sex hormone) and testosterone (a male sex hormone). Notice how subtle differences in structure can cause drastic changes in biological activity. The ketone functionality also shows up in the anti-inflammatory steroid, Cortisone.
Figure 9.11 Examples of aldehyde and ketone containing molecules isolated from plant sources.
Figure 9.12 Examples of aldehyde and ketone containing molecules isolated from animal sources.
To Your Health: Ketones in Blood, Urine, and Breath
Ketones are formed in the human body as a by-product of lipid metabolism. The two common metabolites produced in humans are the ketone-containing, acetoacetic acid and the alcohol metabolite, β-hydroxybutyrate. Acetone is also produced as a breakdown product of acetoacetic acid. Acetone can then be excreted from the body through the urine or as a volatile product through the lungs.
Normally, ketones are not released into the bloodstream in appreciable amounts. For example, the normal concentration of acetone in the human body is less than 1 mg/100 mL of blood. Instead, ketones that are produced during lipid metabolism inside cells are usually fully oxidized and broken down to carbon dioxide and water. This is because glucose is the primary energy source for the body, especially for the brain. Glucose is released in controlled amounts into the bloodstream by the liver, where it travels throughout the body to provide energy. For the brain, this is the primary energy source, as the blood-brain barrier blocks the transport of large lipid molecules. However, during times of starvation, when glucose is unavailable, or in certain disease states where glucose metabolism is disregulated, like uncontrolled diabetes mellitus, the ketone concentrations within blood rises to higher levels to provide an alternative energy source for the brain. However, since acetoacetic acid and β-hydroxybutyrate contain carboxylic acid functional groups, the addition of these molecules to the blood cause acidification which if left unchecked can cause a dangerous condition called ketoacidosis. Ketoacidosis can be a life threatening event. Ketones can be easily detected, as acetone is excreted in the urine. In severe cases, the odor of acetone can also be noted on the breath.
9.5 Carboxylic Acids and Esters
Carboxylic Acids can be easily recognized as they have a carbonyl carbon that is also linked directly to an alcohol functional group. So the carbonyl carbon is also attached directly to an alcohol. In the ester functional group, the carbonyl carbon is also directly attached as part of an ether functional group.
Properties of Carboxylic Acids and Esters
Carboxylic Acids
Carboxylic acids are organic compounds which incorporate a carboxyl functional group, CO2H. The name carboxyl comes from the fact that a carbonyl and a hydroxyl group are attached to the same carbon.
Carboxylic acids are named such because they can donate a hydrogen to produce a carboxylate ion. The factors which affect the acidity of carboxylic acids will be discussed later.
Esters
An ester is an organic compound that is a derivative of a carboxylic acid in which the hydrogen atom of the hydroxyl group has been replaced with an alkyl group. The structure is the product of a carboxylic acid (the R-portion) and an alcohol (the R′-portion). The general formula for an ester is shown below.
The R group can either be a hydrogen or a carbon chain. The R′ group must be a carbon chain since a hydrogen atom would make the molecule a carboxylic acid. The steps for naming esters along with two examples are shown below.
Boiling Points, Melting Points and Solubility
Carboxylic acids can form hydrogen bond dimers which increases their boiling points above that of alcohols of similar size (Table 9.3).
Table 9.3 Comparison of Boiling Points for Compounds of Similar Size
Small esters have boiling points which are lower than those of aldehydes and ketones with similar mass (Table 9.3). Esters, like aldehydes and ketones, are polar molecules. however, their dipole-dipole interactions are weaker than that of aldehydes and ketones and they are unable to form hydrogen bonds. Thus, their boiling points are higher than ethers and lower than aldehydes and ketones of similar size.
Low molecular weight carboxylic acids tend to be liquids at room temperature, whereas larger molecules form waxy solids. Carboxylic acids that range in carbon chain length from 12 -20 carbons are typically called fatty acids, as they are commonly found in fats and oils. Comparable to other oxygen containing molecules, the short-chain carboxylic acids tend to be soluble in water, due to their ability to form hydrogen bonds. As the carbon chain length increases, the solubility of the carboxylic acid in water goes down. Esters can also hydrogen bond with water, although not as efficiently as carboxylic acids, and thus they are slightly less soluble in water than carboxylic acids of similar size.
Carboxylic acids typically have in unpleasant, pungent and even rancid odors. The smell of vinegar, for example, is due to ethanoic acid (also known as acetic acid). The odor of gyms and unwashed socks is largely caused by butanoic acid, and hexanoic acid is responsible for the strong odor of limburger cheese. Due to their acidic nature, carboxylic acids also have a sour taste as noted for vinegar and the citric acid found in many fruits. Esters, on the otherhand, have enjoyable aromas and are responsible for the aroma of many fruits and flowers. Esters can also have fruity flavors.
Practice Problems:
-
Which compound has the higher boiling point—CH3CH2CH2OCH2CH3 or CH3CH2CH2COOH? Explain.
-
Which compound has the higher boiling point—CH3CH2CH2CH2CH2OH or CH3CH2CH2COOH? Explain.
-
Which compound is more soluble in water—CH3COOH or CH3CH2CH2CH3? Explain.
-
Which compound is more soluble in water—CH3CH2COOH or CH3CH2CH2CH2CH2COOH? Explain.
Answers
-
CH3CH2CH2COOH because of hydrogen bonding (There is no intermolecular hydrogen bonding with CH3CH2CH2OCH2CH3.)
-
CH3COOH because it engages in hydrogen bonding with water (There is no intermolecular hydrogen bonding with CH3CH2CH2CH3.)
Commonly Used Carboxylic Acids and Esters
Carboxylic acids and esters are common in nature and are used for a multitude of purposes. For example, ants in the Formicidae family use the simplest carboxylic acid, formic acid, as both a chemical defense and as an attack to subdue prey (Figure 9.13A). A dilute solution of acetic acid (5%) is found in vinegar and is responsible for the sour and pungent flavor. Acetic acid also gives sourdough bread its sharp taste and accounts for the sour flavors in wine. Citric acid is found in many fruits and accounts for their sour flavor. Other carboxylic acids such as PABA and glycolic acid are used in the cosmetic industry. PABA which is produced by plants, fungi, and bacteria is a common component of food and is related in structure to the vitamin folate. In 1943 PABA was patented as one of the first compounds used in the manufacture of sunscreen. However, its use has fallen out of favor since the mid-1980s due to concerns that it may increase cellular UV damage, as well as contribute to allergies. Glycolic acid is the smallest of the acid class known as the α-hydroxy acids, and has found use in both the food processing and cosmetic industry. In food processing it is used as a preservative and in the skin care industry it is used most often as a chemical peel to reduce facial scarring by acne.
Figure 9.13 Sources and uses of common carboxylic acids. (A) Formic acid is the defense toxin used by ants in the Formicidae family. Photo byMuhammad Mahdi Karim (B) Vinegar is a 5% solution of acetic acid. The photo on the left shows various flavors of vinegar at a market in France. Photo byGeorges Seguin (C) Citric acid is a common component of fruit, providing a sour taste. Photo of lemons byAndré Karwath (D) para-Aminobenzoic Acid (PABA) is a carboxylic acid commonly found in plants and food crops including whole grain. It was patented in 1943 for its use in sunscreen products. However, due to safety concerns and allergic response, the use of PABA has been discontinued for this purpose. Suncreen photo provided by HYanWong (E) Glycolic acid is commonly used in cosmetics as a chemical peel used to reduce scarring by acne.in the photo on the left is the before treatment and on the right after several glycolic acid treatments. Glycolic study provided byJaishree Sharad.
Esters are readily synthesized and naturally abundant contributing to the flavors and aromas in many fruits and flowers. For example, the ester, methyl salicylate is also known as the oil of wintergreen (Figure 9.14). The fruity aroma of pineapples, pears and strawberries are caused by esters, as well as the sweet aroma of rum.
Figure 9.14 The Fruity and Pleasant Aromas of Esters can be found in (A) the oil of wintergreen, (B) the aroma of pineapples, and (C) the sweetness of rum. Photo of (A) Gaultheria procumbens, the producer of the oil of wintergreen is provided by: LGPL (B) pineapple photo provided by:David Monniaux, and (C) Photo of rum provided by:Summerbl4ck
Esters also make up the bulk of animal fats and vegetable oils as triglycerides. The formation of lipids and fats will be described in more detail in Chapter 11.
9.6 Reactions of Oxygen-Containing Compounds
Alcohols
Alcohol functional groups can be involved in several different types of reactions. In this section, we will discuss two major types of reactions. The first are the dehydration reactions and the second are the oxidation reactions. Alcohols can also be involved in addition and substitution reactions with other functional groups like aldehydes, ketones, and carboxylic acids. These types of reactions will be discussed in more detail within the aldehyde and ketone, and carboxylic acid sections.
Dehydration (Elimination) Reactions
In chapter 8, we learned that alcohols can be formed from the hydration of alkenes during addition reactions. We also learned that the opposite reaction can also occur. Alcohols can be removed or eliminated from molecules through the process of dehydration (or the removal of water). The result of the elimination reaction is the creation of an alkene and a molecule of water.

Elimination reactions that occur with more complex molecules can result in more than one possible product. In these cases, the alkene will form at the more substituted position (at the carbon that has more carbon atoms and less hydrogen atoms attached to it). For example, in the reaction below, the alcohol is not symmetrical. Thus, there are two possible products of the elimination reaction, option 1 and option 2. In option 1, the alkene is formed with the carbon that has the fewest hydrogens attached, whereas in option 2 the alkene is formed with the carbon that has the most hydrogens attached. Thus, option 1 will be the major product of the reaction and option 2 will be the minor product.
Alcohol elimination reactions using small 1o alcohols can also be used to produce ethers. To produce an ether rather than the alkene, the temperature of the reaction must be reduced and the reaction must be done with excess alcohol in the reaction mixture. For example:
2 CH3CH2-OH + H2SO4 | 130 ºC![]() |
CH3CH2-O-CH2CH3 + H2O |
CH3CH2-OH + H2SO4 | 150 ºC![]() |
CH2=CH2 + H2O |
In this reaction alcohol has to be used in excess and the temperature has to be maintained around 413 K. If alcohol is not used in excess or the temperature is higher, the alcohol will preferably undergo dehydration to yield alkene. The dehydration of secondary and tertiary alcohols to get corresponding ethers is unsuccessful as alkenes are formed too easily in these reactions.
Oxidation Reactions
Some alcohols can also undergo oxidation reactions. Remember in redox reactions, the component of the reaction that is being oxidized is losing electrons (LEO) while the molecule receiving the electrons is being reduced (GER). In organic reactions, the flow of the electrons usually follows the flow of the hydrogen atoms. Thus, the molecule losing hydrogens is typically also losing electrons and is the oxidized component. The molecule gaining electrons is being reduced. For alcohols, both primary and secondary alcohols can be oxidized. Tertiary alcohols, on the other hand, cannot be oxidized. In many oxidation reactions the oxidizing agent is shown above the reaction arrow as [O]. The oxidizing agent can be a metal or another organic molecule. In the reaction, the oxidizing agent is the molecule that is reduced or accepts the electrons.
In alcohol oxidation reactions, the hydrogen from the alcohol and a hydrogen that is attached to the carbon that has the alcohol attached, along with their electrons, are removed from the molecule by the oxidizing agent. Removal of the hydrogens and their electrons results in the formation of a carbonyl functional group. In the case of a primary alcohol, the result is the formation of an aldehyde. In the case of a secondary alcohol, the result is the formation of a ketone. Note that for a tertiary alcohol, that the carbon attached to the alcohol functional group does not have a hydrogen atom attached to it. Thus, it cannot undergo oxidation. When a tertiary alcohol is exposed to an oxidizing agent, no reaction will occur.
Notice that for the primary alcohol that undergoes oxidation, that it still retains a hydrogen atom that is attached to the carbonyl carbon in the newly formed aldehyde. This molecule can undergo a secondary oxidation reaction with an oxidizing agent and water, to add another oxygen atom and remove the carbonyl hydrogen atom. This results in the formation of a carboxylic acid.
To Your Health: The Physiological Effects of Alcohols
Methanol is quite poisonous to humans. Ingestion of as little as 15 mL of methanol can cause blindness, and 30 mL (1 oz) can cause death. However, the usual fatal dose is 100 to 150 mL. The main reason for methanol’s toxicity is that we have liver enzymes that catalyze its oxidation to formaldehyde, the simplest member of the aldehyde family:

Formaldehyde reacts rapidly with the components of cells, coagulating proteins in much the same way that cooking coagulates an egg. This property of formaldehyde accounts for much of the toxicity of methanol.
Organic and biochemical equations are frequently written showing only the organic reactants and products. In this way, we focus attention on the organic starting material and product, rather than on balancing complicated equations.
Ethanol is oxidized in the liver to acetaldehyde:

The acetaldehyde is in turn oxidized to acetic acid (HC2H3O2), a normal constituent of cells, which is then oxidized to carbon dioxide and water. Even so, ethanol is potentially toxic to humans. The rapid ingestion of 1 pt (about 500 mL) of pure ethanol would kill most people, and acute ethanol poisoning kills several hundred people each year—often those engaged in some sort of drinking contest. Ethanol freely crosses into the brain, where it depresses the respiratory control center, resulting in failure of the respiratory muscles in the lungs and hence suffocation. Ethanol is believed to act on nerve cell membranes, causing a diminution in speech, thought, cognition, and judgment.
Rubbing alcohol is usually a 70% aqueous solution of isopropyl alcohol. It has a high vapor pressure, and its rapid evaporation from the skin produces a cooling effect. It is toxic when ingested but, compared to methanol, is less readily absorbed through the skin.
Example Problem:
Write an equation for the oxidation of each alcohol. Use [O] above the arrow to indicate an oxidizing agent. If no reaction occurs, write “no reaction” after the arrow.
- CH3CH2CH2CH2CH2OH
-
-
Solution
The first step is to recognize the class of each alcohol as primary, secondary, or tertiary.
-
This alcohol has the OH group on a carbon atom that is attached to only one other carbon atom, so it is a primary alcohol. Oxidation forms first an aldehyde and further oxidation forms a carboxylic acid.
-
This alcohol has the OH group on a carbon atom that is attached to three other carbon atoms, so it is a tertiary alcohol. No reaction occurs.
-
This alcohol has the OH group on a carbon atom that is attached to two other carbon atoms, so it is a secondary alcohol; oxidation gives a ketone.
More Practice:
Write an equation for the oxidation of each alcohol. Use [O] above the arrow to indicate an oxidizing agent. If no reaction occurs, write “no reaction” after the arrow.
Aldehydes and Ketones
In this section, we will discuss the primary reactions of aldehydes and ketones. These include oxidation and reduction reactions, and combination reactions with alcohols.
Oxidation Reactions
As shown above in the alcohol section, aldehydes can undergo oxidation to produce a coarboxylic acid. This is because the carbonyl carbon atom still retains a hydrogen atom that can be removed and replaced with an oxygen atom. Ketones on the other hand, do not contain a hydrogen atom bound to the carbonyl carbon atom. Thus, they cannot undergo further oxidation. As noted above, ketones that are exposed to an oxidizing agent will have no reaction.
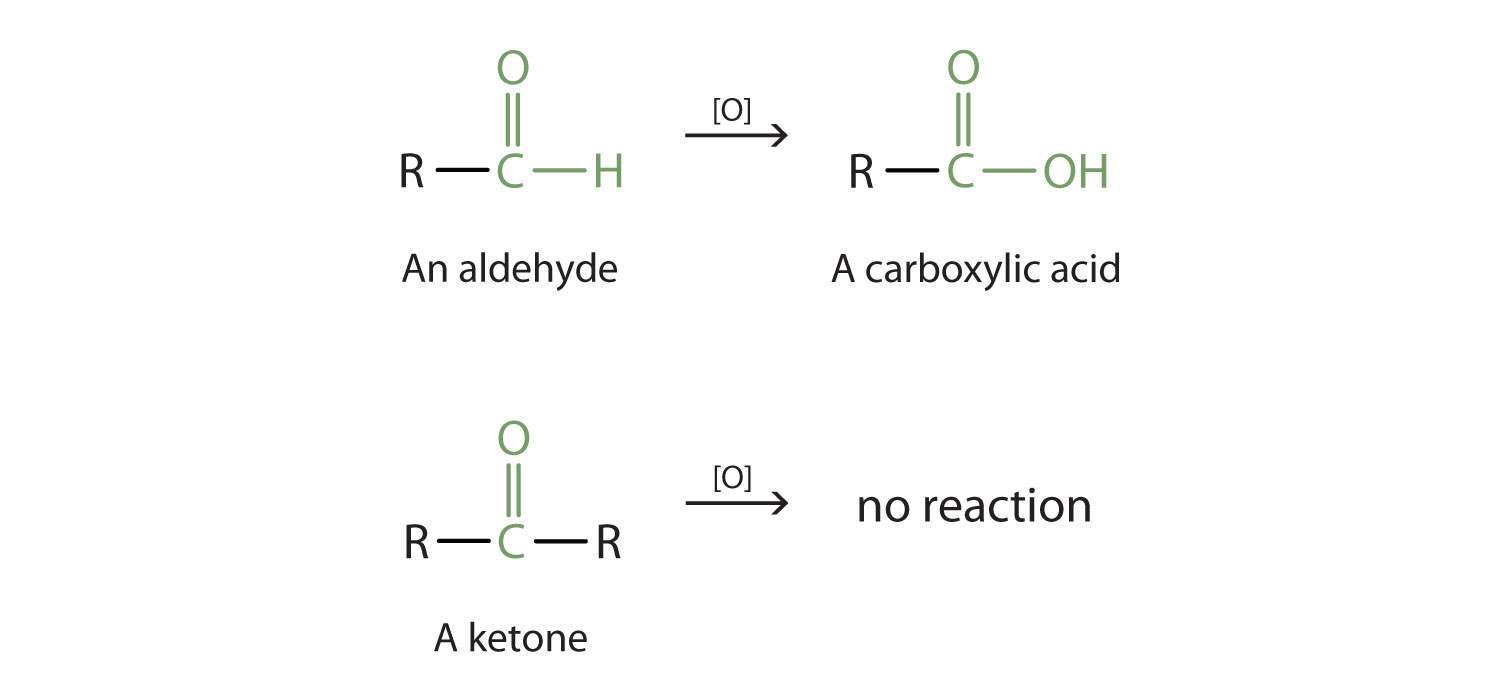
Reduction Reactions
Reduction reactions with aldehydes and ketones revert these compounds to primary alcohols in the case of aldehydes and secondary alcohols in the case of ketones. They are essentially the reverse reactions of the alcohol oxidation reactions.
For example, with the aldehyde, ethanal you get primary alcohol, ethanol:
Notice that this is a simplified equation where [H] means “hydrogen from a reducing agent”. In general terms, reduction of an aldehyde leads to a primary alcohol.
Reduction of a ketone, such as propanone will give you a secondary alcohol, such as 2-propanol:
Reduction of a ketone leads to a secondary alcohol.
Addition Reactions with Alcohols
Aldehydes and ketones can react with alcohol functional groups in addition (combination) reactions. These types of reactions are common in nature and are very important in the cyclization process of sugar molecules. We will return to this subject in chapter 11 in our introduction to the major macromolecules of the body.
When an alcohol adds to an aldehyde, the result is called a hemiacetal; when an alcohol adds to a ketone the resulting product is a hemiketal.
In the reaction above, the B: is referring to a general base that is present in the solution and can act as a proton acceptor. In this reaction, a general base activates the alcohol in the reaction (the oxygen of the alcohol is shown in red). The oxygen of the alcohol is then negatively charged, because it is carrying the extra electron from the hydrogen. It can now act as a nucleophile and attack the carbonyl carbon of the aldehyde or ketone. When the oxygen of the alcohol forms a bond with the carbonyl carbon of the aldehyde or ketone, this displaces one of the double bonds of the carbonyl group. The oxyen from the carbonyl will then pull a hydrogen from a general acid that is present in the solution. In this diagram, the general acid is shown as H-A. This forms an alcohol where the carbonyl group of the aldehyde or ketone used to be. The original alcohol group now looks like an ether functional group. So you can recognize hemiacetals and hemiketals in natural products as a carbon atom that is bonded to both an alcohol and an ether functional group at the same time. If that carbon also has hydrogen bonded to it, it originated from the aldehyde and is termed the hemiacetal. If the central carbon is bonded to two other carbon atoms (designeated R1 and R3 above) in addition to the oxygen atoms, the molecule originated from a ketone and it is called the hemiketal.
The prefix ‘hemi’ (half) is used in each term because, as we shall soon see, a second addition of an alcohol nucleophile can occur, resulting in species called acetals and ketals.
The formation of hemiacetals and hemiketals within biological systems is common and often occurs spontaneously (without a catalyst or enzyme present), especially in the case of simple sugar molecules. Due to the spontaneity of the reactions, they are also highly reversible: hemiacetals and hemiketals easily convert back to aldehydes and ketones plus alcohol. The mechanism for the conversion of a hemiacetal back to an aldehyde is shown below:
Practice Problems:
Reactions that form Acetals or Ketals
When a hemiacetal (or hemiketal) is subjected to nucleophilic attack by a second alcohol molecule, the result is called an acetal (or ketal).
While the formation of a hemiacetal from an aldehyde and an alcohol (step 1 above) is a nucleophilic addition, the formation of an acetal from a hemiacetal (step 2 above) is a nucleophilic substitution reaction, with the original carbonyl oxygen (shown in blue) leaving as a water molecule. Since water is leaving the molecule in the second reaction (step 2), this reaction is also known as a dehydration reaction. The substitution reaction occurring in the second step does not happen spontaneously and is not easily reversible. Inside biological systems, an enzyme would be required for the formation of an acetal or ketal. Note that the acetal and ketal both look like a central carbon bonded to two ether functional groups. If that central carbon is also bonded to a hydrogen, then it is the acetal, and if it is bonded to two carbons, it is the ketal. The reverse reaction would involve the breakdown of the acetal or ketal using hydrolysis or the entry of water into the molecule.
Practice Problems:
Carboxylic Acids
The Acidity of Carboxylic Acids
Using the definition of an acid as a “substance which donates protons (hydrogen ions) to other things”, the carboxylic acids are acidic because the hydrogen in the -COOH group can be donated to other molecules. In solution in water, a hydrogen ion is transferred from the -COOH group to a water molecule. For example, with ethanoic acid (as shown below), you get an ethanoate ion formed together with a hydronium ion, H3O+.
This reaction is reversible and, in the case of ethanoic acid (acetic acid), no more than about 1% of the acid has reacted to form ions at any one time.
Thus, carboxylic acids are weak acids.
Carboxylic Acids and the Formation of Salts
Due to their acidic nature, carboxylic acids can react with the more reactive metals to form ionic bonds and create salts. The reactions are just the same as with acids like hydrochloric acid, except they tend to be rather slower.
2CH3COOH(aq) + Mg(s) → (CH3COO)2Mg + H2
In the reaction above, dilute ethanoic acid reacts with magnesium. The magnesium reacts to produce a colorless solution of magnesium ethanoate, and hydrogen gas is given off. If you use magnesium ribbon, the reaction is less vigorous than the same reaction with hydrochloric acid, but with magnesium powder, both are so fast that you probably wouldn’t notice much difference.
Example Problem:
Write an equation for each reaction.
- the ionization of propionic acid (CH2CH2COOH) in water (H2O)
- the neutralization of propionic acid with aqueous sodium hydroxide (NaOH)
Solution:
-
Propionic acid ionizes in water to form a propionate ion and a hydronium (H3O+) ion.
CH3CH2COOH(aq) + H2O(ℓ) → CH3CH2COO−(aq) + H3O+(aq)
-
Propionic acid reacts with NaOH(aq) to form sodium propionate and water.
CH3CH2COOH(aq) + NaOH(aq) → CH3CH2COO−Na+(aq) + H2O(ℓ)
Formation of Esters from Carboxylic Acids and Alcohols
An ester can be formed by combining a carboxylic acid with an alcohol in the presence of a strong acid, or in the presence of an enzyme, if in biological systems. In the esterification reaction, the hydroxyl group of the carboxylic acid acts as a leaving group and forms the water molecule in the final product. It is replaced by the -OR group from the alcohol.
The reaction is reversible. As a specific example of an esterification reaction, butyl acetate can be made from acetic acid and 1-butanol.
A Closer Look: Condensation Polymers
A commercially important esterification reaction is condensation polymerization, in which a reaction occurs between a dicarboxylic acid and a dihydric alcohol (diol), with the elimination of water. Such a reaction yields an ester that contains a free (unreacted) carboxyl group at one end and a free alcohol group at the other end. Further condensation reactions then occur, producing polyester polymers.
The most important polyester, polyethylene terephthalate (PET), is made from terephthalic acid and ethylene glycol monomers:
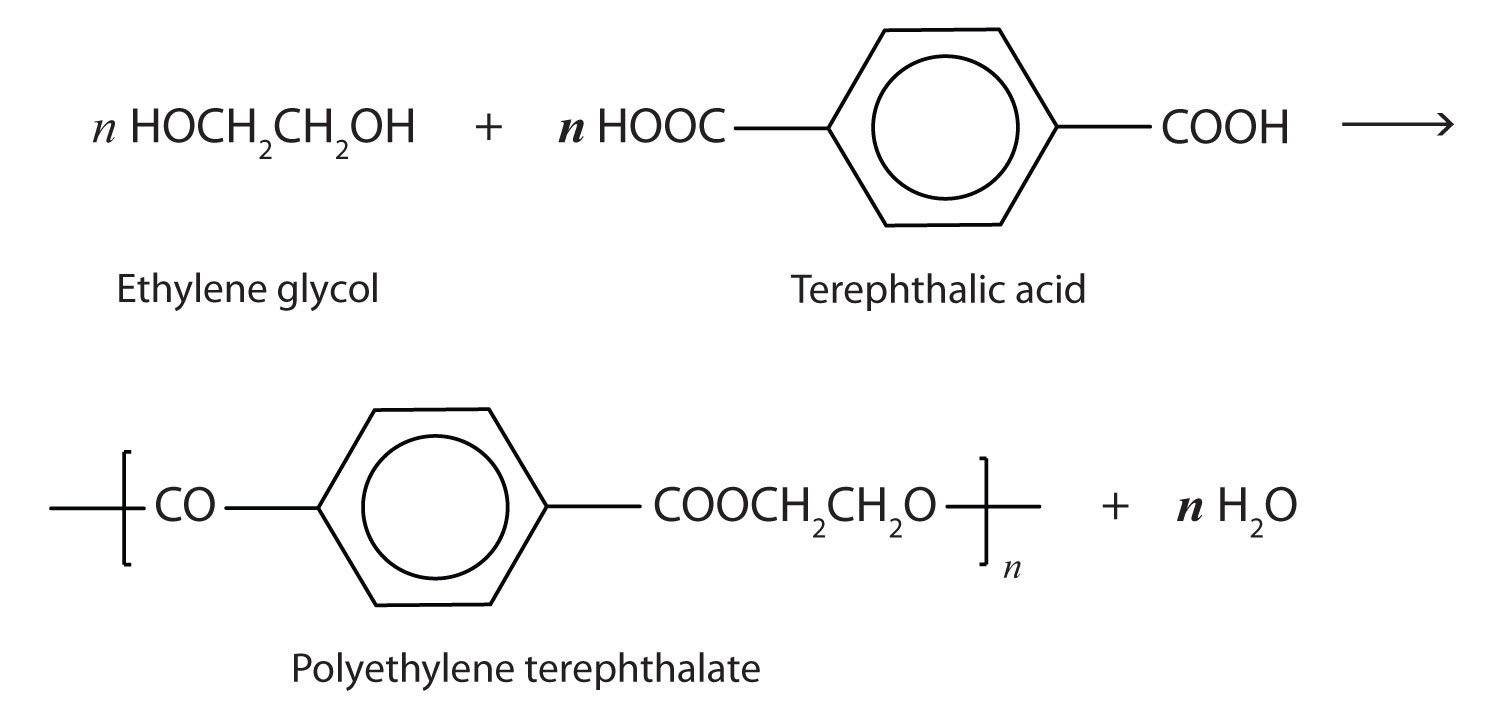
Polyester molecules make excellent fibers and are used in many fabrics. A knitted polyester tube, which is biologically inert, can be used in surgery to repair or replace diseased sections of blood vessels. PET is used to make bottles for soda pop and other beverages. It is also formed into films called Mylar. When magnetically coated, Mylar tape is used in audio- and videocassettes. Synthetic arteries can be made from PET, polytetrafluoroethylene, and other polymers.
Practice Problems:
Complete the Following Reactions:
Hydrolysis of Esters
The reverse reaction of ester formation can be used to breakdown esters into a carboxylic acid and an alcohol. This reactions requires the incorporation of water into the ester linkage, and is thus called a hydrolysis reaction.
The ester is heated with a large excess of water containing a strong-acid catalyst. Like esterification, the reaction is reversible and does not go to completion.

As a specific example, butyl acetate and water react to form acetic acid and 1-butanol. The reaction is reversible and does not go to completion.

Practice Problems:
9.7 Chapter Summary
The hydroxyl group (OH) is the functional group of the alcohols. The alcohols are represented by the general formula ROH. Alcohols are derived from alkanes by replacing one or more hydrogen atoms by an OH group. A primary (1°) alcohol (RCH2OH) has the OH group on a carbon atom attached to one other carbon atom; a secondary (2°) alcohol (R2CHOH) has the OH group on a carbon atom attached to two other carbon atoms; and a tertiary (3°) alcohol (R3COH) has the OH group on a carbon atom attached to three other carbon atoms.
The ability to engage in hydrogen bonding greatly increases the boiling points of alcohols compared to hydrocarbons of comparable molar mass. Alcohols can also engage in hydrogen bonding with water molecules, and those with up to about four carbon atoms are soluble in water.
Many alcohols can be synthesized by the hydration of alkenes. Common alcohols include methanol, ethanol, and isopropyl alcohol. Methanol is quite poisonous. It can cause blindness or even death. Ethanol can be prepared from ethylene or made by fermentation. It is the “alcohol” in alcoholic beverages. On occasion, people drink methanol by mistake, thinking it is the beverage alcohol. On occasion, unscrupulous bootleggers, sell methanol to unsuspecting customers. In either case, the results are often tragic.
When water is removed from an alcohol in a dehydration step, the result is either an alkene or an ether, depending on the reaction conditions. Primary alcohols are oxidized to aldehydes or carboxylic acids, and secondary alcohols are oxidized to ketones. Tertiary alcohols are not easily oxidized.
Alcohols containing two OH groups on adjacent carbon atoms are called glycols.
Phenols (ArOH) are compounds having the OH group attached to an aromatic ring.
Ethers (ROR′, ROAr, ArOAr) are compounds in which an oxygen atom is joined to two organic groups. Ether molecules have no OH group and thus no intermolecular hydrogen bonding. Ethers therefore have quite low boiling points for a given molar mass. Ether molecules have an oxygen atom and can engage in hydrogen bonding with water molecules. An ether molecule has about the same solubility in water as the alcohol that is isomeric with it.
The carbonyl group, a carbon-to-oxygen double bond, is ubiquitous in biological compounds. It is found in carbohydrates, fats, proteins, nucleic acids, hormones, and vitamins—organic compounds critical to living systems.
The carbonyl group is the defining feature of aldehydes and ketones. In aldehydes at least one bond on the carbonyl group is a carbon-to-hydrogen bond; in ketones, both available bonds on the carbonyl carbon atom are carbon-to-carbon bonds. Aldehydes are synthesized by the oxidation of primary alcohols. The aldehyde can be further oxidized to a carboxylic acid. Ketones are prepared by the oxidation of secondary alcohols. Mild oxidizing agents oxidize aldehydes to carboxylic acids. Ketones are not oxidized by these reagents.
Aldehydes and ketones can react with alcohols to form hemiacetals and hemiketals, respectively. These reactions occur without the addition of a catalyst and can move in both the forward and reverse directions. Hemiacetals and hemiketals can go on to react with an additional alcohol molecule to form acetals and ketals. The formation of the acetal or ketal requires the removal of water and is called a dehydration reaction. These reactions require a catalyst or enzyme to cause them to happen. The reverse reaction that breaks apart acetal to form the hemiacetal and the alcohol, requires the addition of a water molecule and is called hydrolysis.
A carboxylic acid (RCOOH) contains the functional group COOH, called the carboxyl group, which has an OH group attached to a carbonyl carbon atom. An ester (RCOOR′) has an OR′ group attached to a carbonyl carbon atom.
A carboxylic acid is formed by the oxidation of an aldehyde with the same number of carbon atoms. Because aldehydes are formed from primary alcohols, these alcohols are also a starting material for carboxylic acids.
Carboxylic acids have strong, often disagreeable, odors. They are highly polar molecules and readily engage in hydrogen bonding, so they have relatively high boiling points.
Carboxylic acids are weak acids. They react with bases to form salts and with carbonates and bicarbonates to form carbon dioxide gas and the salt of the acid.
Esters are pleasant-smelling compounds that are responsible for the fragrances of flowers and fruits. They have lower boiling points than comparable carboxylic acids because, even though ester molecules are somewhat polar, they cannot engage in hydrogen bonding. However, with water, esters can engage in hydrogen bonding; consequently, the low molar mass esters are soluble in water. Esters can be synthesized by esterification, in which a carboxylic acid and an alcohol are combined under acidic conditions. Esters are neutral compounds that undergo hydrolysis, a reaction with water. Under acidic conditions, hydrolysis is essentially the reverse of esterification.
Figure 9.15 Summary of Important Reactions with Oxygen.
9.8 References:
Farmer, S., Reusch, W., Alexander, E., and Rahim, A. (2016) Organic Chemistry. Libretexts. Available at: https://chem.libretexts.org/Core/Organic_Chemistry
Ball, et al. (2016) MAP: The Basics of GOB Chemistry. Libretexts. Available at: https://chem.libretexts.org/Textbook_Maps/Introductory_Chemistry_Textbook_Maps/Map%3A_The_Basics_of_GOB_Chemistry_(Ball_et_al.)/14%3A_Organic_Compounds_of_Oxygen/14.10%3A_Properties_of_Aldehydes_and_Ketones
McMurray (2017) MAP: Organic Chemistry. Libretexts. Available at: https://chem.libretexts.org/Textbook_Maps/Organic_Chemistry_Textbook_Maps/Map%3A_Organic_Chemistry_(McMurry)
Soderburg (2015) Map: Organic Chemistry with a Biological Emphasis. Libretexts. Available at: https://chem.libretexts.org/Textbook_Maps/Organic_Chemistry_Textbook_Maps/Map%3A_Organic_Chemistry_With_a_Biological_Emphasis_(Soderberg)
Antifreeze. (2017, January 5). In Wikipedia, The Free Encyclopedia. Retrieved 06:07, April 21, 2017, from https://en.wikipedia.org/w/index.php?title=Antifreeze&oldid=758484047
Ethylene glycol. (2017, April 4). In Wikipedia, The Free Encyclopedia. Retrieved 06:09, April 21, 2017, from https://en.wikipedia.org/w/index.php?title=Ethylene_glycol&oldid=773769112